Introduction
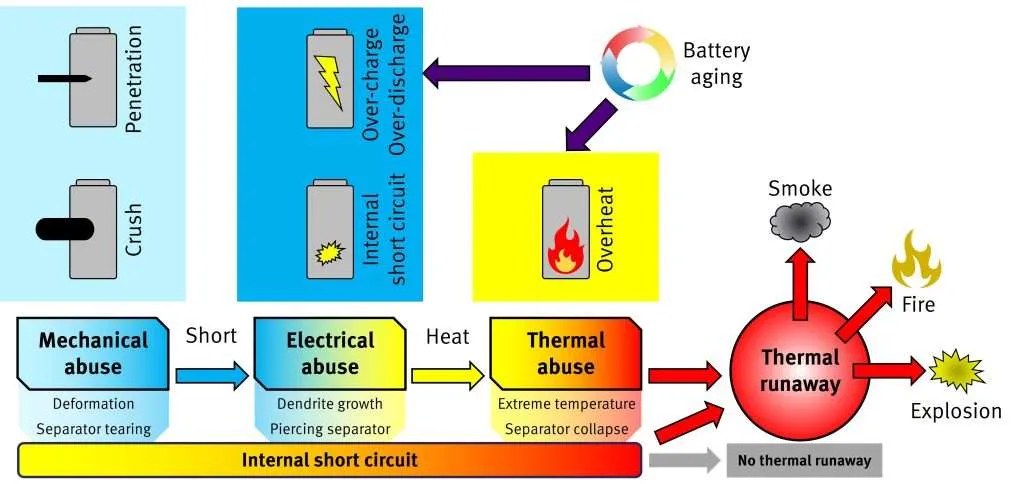
As the demand for electric vehicles (EVs) and renewable energy storage solutions continues to surge, ensuring the safety and reliability of batteries is paramount. One critical aspect of battery performance is understanding and mitigating the risks associated with thermal runaway. In this article, we delve into the world of Battery Thermal Runway Analysis, shedding light on its significance and practical implications.
The Significance of Thermal Runaway in Batteries
Thermal runaway refers to a self-sustaining, uncontrollable increase in temperature within a battery cell, potentially leading to catastrophic failure. Understanding the conditions and triggers that can lead to thermal runaway is crucial for designing safe and reliable battery systems. We explore the science behind thermal runaway and its implications for battery safety.
● Understanding Thermal Runaway: Chain Reactions Leading to Uncontrolled Heating
● Safety Concerns: The Potential Hazards of Thermal Runaway in Battery Systems
● Causes and Triggers: Overcharging, Overheating, and Physical Damage
● High-Energy-Density Batteries: Increased Risk and Importance of Runaway Mitigation
● Impact on Battery Lifespan: Thermal Runaway’s Effect on Battery Degradation
● Containment and Venting Systems: Engineering Safeguards against Thermal Runaway
● Fire Suppression: Measures to Control Fires Resulting from Thermal Runaway
● Thermal Management Strategies: Cooling and Heat Dissipation to Prevent Overheating
● Testing and Certification: Ensuring Battery Safety under Extreme Conditions
● Industry Regulations: Compliance and Standards for Safe Battery Design and Usage.
Battery Components and Thermal Runway Risks
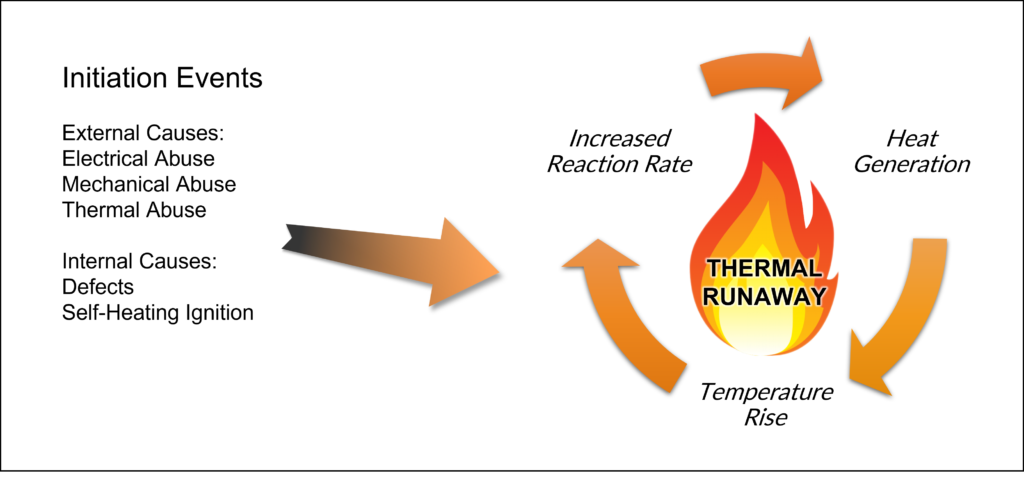
Different components within a battery cell, such as the electrodes, electrolyte, and separators, each have specific thermal thresholds and behaviors. This section provides an in-depth understanding of how these components interact and how their thermal properties contribute to the risk of thermal runaway. By comprehending these factors, engineers can implement measures to mitigate risks.
Battery Chemistries and Thermal Runway
Various battery chemistries, such as lithium-ion, lithium-polymer, and solid-state batteries, have different thermal characteristics and risks associated with them. Understanding the specific attributes of each chemistry is crucial for designing appropriate thermal management systems.
We’ll delve into how different chemistries impact the potential for thermal runaway.
● Lithium-Ion (Li-ion) Batteries: Widely Used in Portable Electronics and Electric Vehicles
● Nickel-Cadmium (Ni-Cd) Batteries: Known for Durability but Less Common Today
● Nickel-Metal Hydride (Ni-MH) Batteries: A Middle Ground in Performance and Cost
● Lead-Acid Batteries: Traditional and Reliable, Commonly Found in Automotive Applications
● Lithium Polymer (Li-Po) Batteries: Lighter and More Flexible than Traditional Li-ion
● Solid-State Batteries: Next-Generation Technology with Enhanced Safety and Energy Density
● Thermal Runaway in Li-ion Batteries: Causes, Triggers, and Chain Reaction Effects
● Sensitivity to Temperature Extremes: How Battery Chemistry Affects Thermal Stability
● Strategies for Mitigating Thermal Runaway: From Improved Materials to Active Cooling
● Future Directions: Advancements in Battery Chemistries for Enhanced Thermal Safety.
Factors Influencing Thermal Runway
Several external factors can influence the likelihood of thermal runaway, including ambient temperature, charge/discharge rates, and mechanical stresses. This section explores how these variables can contribute to thermal runaway events and emphasizes the importance of comprehensive analysis to account for all potential scenarios.
Testing and Simulation Techniques for Thermal Runway Analysis
Advanced testing methods, such as Accelerating Rate Calorimetry (ARC) and Differential Scanning Calorimetry (DSC), are used to analyze the thermal behavior of batteries under controlled conditions. Additionally, computational simulations and modeling allow engineers to predict and understand the potential for thermal runaway in different scenarios. We’ll explore how these techniques are employed in Thermal Runway Analysis.
● Thermal Testing Methods: Calorimetry, Differential Scanning Calorimetry (DSC), and Accelerating Rate Calorimetry (ARC)
● Adiabatic Calorimetry: Evaluating Heat Generation and Heat Transfer in Batteries
● Electrochemical Impedance Spectroscopy (EIS): Assessing Battery Performance and Stability
● Abuse Testing: Simulating Extreme Conditions to Evaluate Thermal Runaway Risk
● Finite Element Analysis (FEA): Numerical Simulation for Predicting Heat Generation and Dissipation
● Computational Fluid Dynamics (CFD): Analyzing Heat Transfer and Fluid Flow in Battery Systems
● Thermal Modeling: Creating Predictive Models to Assess Thermal Runaway Potential
● Battery Management Systems (BMS): Implementing Monitoring and Safety Measures
● Failure Modes and Effects Analysis (FMEA): Identifying Weaknesses in Battery Design
● Environmental Chambers: Controlled Environments for Testing Battery Performance under Extreme Conditions.
Mitigating Thermal Runway Risks: Thermal Management
Systems
Designing effective thermal management systems is crucial for minimizing the risk of thermal runaway. These systems include methods for controlling temperature, such as passive cooling, active cooling, and phase change materials. This section delves into the strategies and technologies used to regulate battery temperature and prevent thermal runaway.
Future Trends: Innovations in Battery Safety
As battery technology continues to advance, so too will the strategies and technologies for mitigating thermal runaway risks. This section provides a glimpse into future trends, including advancements in materials, coatings, and innovative design concepts that promise to further enhance battery safety.
● Solid-State Battery Technology: Promising Enhanced Safety through Solid Electrolytes
● Advanced Thermal Management Systems: Active Cooling and Heat Dissipation Solutions
● Smart Battery Management Systems (BMS): Real-time Monitoring and Control for Safety
● Self-Healing Materials: Materials that Repair and Restore Structural Integrity
● Multi-layered Safety Approaches: Combining Redundant Safeguards for Fail-Safe Operation
● Fire-Resistant Electrolytes: Minimizing Fire Hazards in Case of Thermal Runaway
● Next-Generation Materials and Coatings: Enhancing Thermal and Electrical Stability
● Artificial Intelligence (AI) Integration: Predictive Analytics for Early Warning Systems
● Redox Flow Batteries: Potentially Safer Energy Storage Solutions for Grid Applications
● Industry Standards and Regulations: Evolving Guidelines for Safe Battery Design and Use.
Conclusion: Safeguarding the Future of Energy Storage
Battery Thermal Runway Analysis is a critical aspect of ensuring the safety and reliability of energy storage solutions. By comprehensively understanding the thermal behavior of batteries and implementing effective thermal management systems, engineers are pioneering safer and more reliable battery technology. As the world transitions towards a more sustainable energy landscape, these advancements in battery safety will play a pivotal role in shaping the future of energy storage.